Features
Nikolaos Farmakidis invents the ‘Nanocalligraphy’ tip.
written by Méadhbh O’Connor, artist in residence.
Pressing a pen to paper, my hand travels like contours over a yet unformed topography. Spheres, globules, waves, lines and arrows emerge; rotating, floating, interacting. ‘...flipping the magnetic spins of atoms in a 2D monolayer,’ a physicist friend had been gabbling down the phone to me. Or something. His voice, converted to signals and bounced from relay to relay over a continent to me in a flash, reconfigures and amplifies out of the device into my ear.
‘...monopoles produced immediately after the big bang,’ another friend casually says in a phone call about something else.

My mind drifts as the words hit my ear, meandering around spaces constructed in my head. Spinning balls, immense spaces, tiny spaces, blackness, light, no space...
‘Less like points and more like a field,’ I recall someone saying. I hold the page up in front of me with the drawing.
The marks project something back, something I don’t know consciously and can’t pin down to any singular meaning. I don’t know what it is, I don’t know what to call it and I don’t know where it came from. And yet the thoughts flowed down my arm and onto a page, like opening a valve into the mind.
To make a mark is creativity in its earliest form. From the child who, as if by instinct, reaches for the crayon to daub their first impressions, to the back of the envelope calculation that leads to a new discovery. In new technologies, the printing of a circuit board - yet another kind of mark making - has opened up the possibility for almost limitless information to be created, computed and stored on the countless gadgets that populate our world today. The mind boggles.
I find myself as an artist working, somehow, with a lively group of engineers at Oxford with the Advanced Nanoscale Engineering group, led by Harish Bhaskaran
Today, I find myself as an artist working, somehow, with a lively group of engineers at Oxford with the Advanced Nanoscale Engineering group, led by Harish Bhaskaran.
Wires, computers, various gadgets, gas canisters, something called an atomic force microscope, containers with tiny chips, stacks of silicon wafers, labels, tools... an abundance of odd objects are scattered around the lab. I see all this stuff and think sculpturally, ‘What could I make with this colourful wire?’
What I see is only the housing. The magic of what the group does is beyond the perception of the naked eye. One nanometer, or 100,000 times smaller than the width of a human hair, is one of the scales they use.
‘We are finding ways to capture the energy of light within nanoscale wires, while still figuring out how to make all of these sustainably and in new exotic formats,’ Professor Bhaskaran tells me.
We are finding ways to capture the energy of light within nanoscale wires, while still figuring out how to make all of these sustainably and in new exotic formats
Professor Bhaskaran
The group is busy creating a new world at this invisible scale. Lab meetings are held every other week. But we converge, instead, inside the very kind of machine their research helps to create: the computer. Transformed into pixels and in our boxes on the Zoom screen, the same questions seem to arise again and again.
‘How can we do this?’
‘What could that mean?’
‘Is there another way?’
‘Why not try this?’
Ideas dash back and forth at speed. One afternoon, I find myself in the lab talking to one or two members of the group. As expected, possibilities and ideas are being discussed with vigour. In all the enthusiastic gesticulations something flies across the room.
‘What was that?’ ‘Nothing...’
I notice the little round case that rolled across the floor with a now cracked, but thankfully unused, silicon wafer on top.
Nikolaos Farmakidis is neatly handwritten across the label. Unaware of the chaos that had just momentarily erupted in the lab, Nik is in his office concentrating fixedly at his computer, as he does every day with unyielding focus.
‘My main interests are trying to bridge optical and electrical methods for computer processing,’ he says, with a humility that belies his futuristic research.
My main interests are trying to bridge optical and electrical methods for computer processing, says Nikolaos Farmakidis. It is a bit different from my usual research. A side project...I designed a new tip for lithography at the nanoscale
He tells me of a paper he’s been working on. One of many, I note.
‘It is a bit different from my usual research. A side project,’ he modestly says.
Something unexpected, I interpret his remark.
‘I designed a new tip for lithography at the nanoscale.’
He shows me an image of an elegant circle, wide at the top and gradually narrowing at its side, and repeating again to close the infinite loop. I learn that I am seeing 100 microns, again, far smaller than the width of a single human hair. My eye darts to the right and another image is on the page, ‘My Life’ scribed beautifully in Arabic.
How better to demonstrate its potential than to consult a calligrapher, Majid Alyousef, a master of the mark. The elegant letters form an ambigram, turned upside down it cleverly reads the same. Much like Nik’s perfect circle. Nano-calligraphy he calls this novel method. Or to be more precise: Nano-Calligraphy Scanning Probe Lithography (nc-SPL). Mechanical nanolithography which can be extended to the patterning of biological materials such as proteins or DNA or even sheets, one atom thick.
A new probe to score the minutest of marks with the atomic-scale movements of the microscope it sits in. Some hobbyists even make their own such machines. It’s an inexpensive new tool for them too.
Nik and his collaborators have fashioned a new tool not just for the researcher experimenting at the frontiers of computing, but also the hobbyist, the artist and the curious to make a new mark on the world
As we stop chatting I turn back to my laptop to continue making pictures, with pixels this time, and realise how much I had taken it for granted. Every chip, screen, logic gate, the circuitry, programming language, the billions of parts, had to be thought up and made by somebody or somebodies. A humming, pulsating, electrical monument to the creativity of countless people. I stare at the device, somehow seeing it anew, and am suddenly jolted out of this distant thought. A ping leaps out of the phone, then the laptop, then the computer: a reminder with ‘Bi-weekly lab meeting’ appears on every screen.
I log in to listen in again. Never resting, the group has moved on to the next burning questions. In the meantime, Nik and his collaborators have fashioned a new tool not just for the researcher experimenting at the frontiers of computing, but also the hobbyist, the artist and the curious to make a new mark on the world.
Méadhbh O’Connor is a visual artist from Ireland who has dedicated her art practice to bringing art and science together. She is artist in residence with the Advanced Nanoscale Engineering group in the Department of Materials, University of Oxford

New research from the University of Oxford, in collaboration with 15 other institutions and NGOs around the globe, outlines the opportunities and challenges associated with organising virtual meetings.
The global pandemic of COVID-19 and associated human-movement restrictions has resulted in mass postponements or cancellations of in-person meetings. An essential part of any academic career, conferences offer a unique opportunity for scientists to interact, network and form partnerships.
'We felt that outlining our lessons learned from organising an online meeting and providing information on actual costs and platforms would be useful to future meeting organisers planning similar events, since online meetings are here to stay.' Dr Paris Stefanoudis, University of Oxford, Zoology Department.
Their absence is likely to impact early-career researchers the most because they have time-sensitive academic paths that rely on conferences to disseminate their work, connect with established researchers and ultimately advance their academic careers.
In this work, published in the Proceedings of the Royal Society B: Biological Sciences, a team of 14 authors describe their experience of organising a medium-sized (<400 participants) international meeting held online in August 2020, and outline the logistics involved in administering such an event. In addition, they present the participants’ perspective, drawn from a post-meeting questionnaire and finally, compare demographic data from this meeting with past, in-person meetings of similar-themed symposia.
'We felt that outlining our lessons learned from organising an online meeting and providing information on actual costs and platforms would be useful to future meeting organisers planning similar events, since online meetings are here to stay. We certainly wish we had that information available when we started organising our meeting.' Dr Paris Stefanoudis, University of Oxford, Zoology Department.
A post-conference questionnaire indicated that participants regarded live talks as a key component of online meetings and were preferred over pre-recorded talks, although most supported the option of having the latter to cater for those with broadband issues or time zone conflicts. Respondents felt that the virtual meeting did not fully replicate the in-person experience, as for example, they were not able to engage with other researchers as much as with past in-person meetings, and received less feedback for their presented work.
‘While we have shown that it is certainly possible to minimise organising costs for online meetings, it is not sustainable in the long run to expect online meetings to be run on a volunteer-basis.’ Dr Paris Stefanoudis, University of Oxford, Zoology Department
In addition, most could not allocate as much time as for in-person meetings and almost half said they were less concentrated. Although not specifically identified in the study, the authors suggest that that personal commitments, time zone differences, online fatigue, and lack of care for dependents - all of which have been augmented during the COVID-19 pandemic – may have played a role in that. Despite those caveats, the vast majority of respondents enjoyed the online meeting and indicated that they would join similar events again.
From a financial perspective, cost for participants as well as for organisers was a fraction of that of in-person meetings. The meeting organisers reduced costs by not hiring a commercial conference company, and instead using ‘off-the-shelf’ software products, largely volunteering their time, and only spending some funds to pay for three researchers to take on coordinating and technical support duties.
‘While we have shown that it is certainly possible to minimise organising costs for online meetings, it is not sustainable in the long run to expect online meetings to be run on a volunteer-basis. It is also not equitable as well, since inevitably this will lead to future online conferences being organised predominantly by researchers in the Global North that have the financial luxury to volunteer their time for free.’
'Going forward a tiered registration or registration waivers should be used to offset costs for those in financial need’ Dr Paris Stefanoudis, University of Oxford, Zoology Department
‘Going forward, online meetings of this size would require hiring more paid organising and technical support staff or a professional company, which would somewhat increase online participating costs. In any case, a tiered registration or registration waivers should be used to offset costs for those in financial need’ Dr Paris Stefanoudis, University of Oxford, Zoology Department
Moreover, the online meetings’ demographic composition compared favourably to that of a similar-themed, past in-person meeting held in a high-income nation in 2018, with the former doubling the number of participants from low and middle-income nations. Interestingly, demographic data were comparable to an in-person meeting held in an upper-middle-income nation, indicating that holding in-person meetings in developing economies can be as effective in widening participation as virtual meetings.
Tardigrades, known colloquially as water bears or moss piglets, are eight-legged segmented micro-animals. There are about 1,300 known species in the phylum Tardigrada, with the earliest known specimens having been found in amber from 145 to 66 million years ago, but it’s likely they have a significantly earlier origin, maybe from over 500 million years ago.
These ‘slow steppers’ are one of the toughest and most resilient form of life on earth, with some species able to survive for up to 30 years without food or water and endure temperature extremes of up to 150 degrees Celsius. Yet they can be found almost anywhere: Tardigrades are prevalent in mosses and lichens and feed on plant cells, algae, and small invertebrates. When collected, they may be viewed under a low-power microscope, making them great for research by students and amateur scientists.
'Tardigrades can survive the deepest seas, artic temperatures and even the frozen vacuum of space'. Dr Jasmine Nirody
Tardigrades are usually about 0.5 mm long when fully grown. Animals as small and soft as tardigrades seldom have legs and almost never bother walking - round worms of similar size and body type thrash about, slithering their doughy forms over unpredictable substrates.
Yet the water bear uses eight stubby legs to improbably propel itself through marine and freshwater sediment, across desert dunes, and beneath the soil. Their dumpy plod raises the question of why tardigrades evolved to walk at all.
They are so small that they generate and feel miniscule inertial forces, so walking in water for them is like a human walking through a much more viscous fluids, like honey. Yet they’re able to plod along like animals over 100,000 times their size.
Recent research, joint between the University of Oxford, Rockefeller University, and Princeton University, found that their coordination mirrors the stepping patterns of larger panarthropods such as stick insects and spiders. Can there be any ‘universal’ locomotive strategies in a set of animals that includes cicadas, lobsters, tardigrades, & centipedes? This diverse group spans an overwhelmingly wide range of sizes, body plans, and habitats.
Unlike vertebrates, which have distinct gaits for each speed - picture a horse's hooves as it transitions from a walk to a gallop - tardigrades run more like insects, scurrying at increasing speeds without ever changing their basic stepping patterns. These stepping patterns seem to follow a small set of 'coordination rules' that pop out when we look at the phase offsets between individual leg pairs - so, for example how soon will the front right leg be lifted after the leg directly behind it.
'Perhaps the best way to navigate unpredictable terrain with a microscopic body is to plod like a water bear.' Dr Jasmine Nirody
Tardigrades also respond to changes in substrate stiffness. As the ground under their feet gets softer and gives way, they switch to a ‘bounding’ coordination pattern. The same switch - from out-of-phase to in-phase movement of leg pairs - has also been observed in desert beetles, which are significantly larger and have rigid bodies - moving along shifting sands.
These observed commonalities may suggest the same low-dimensional control architecture is used across insect taxa, or more generally across panarthropods. This discovery implies the existence of either a common ancestor or an evolutionary advantage that explains why one of the smallest and softest creatures evolved to walk just like larger, hard-bodied panarthropods.
These similarities open up several interesting evolutionary questions. One possible explanation is that tardigrades, long assumed to fit neatly into no existing taxonomy, may share common ancestors - and even a common neural circuit - with arthropods such as fruit flies, ants, spiders, and other segmented scurrying creatures.
One hypothesis for a shared neural architecture is rooted in the structure of the insect ventral nerve cord (VNC), a part of the nervous system that sends out signals to the legs. This structure is well conserved across panarthropods.
Of course, in a group as diverse as panarthropods, there are going to be exceptions to even the most 'universal' rules. There are desert beetles that gallop instead of walking. Some centipedes send ‘backwards’ traveling waves instead of forward. But such divergences from a core set are exciting, not discouraging. They can give us hints into what features of a strategy are important for specialized performance in particular environments.
'We don't know much about what happens at the extremes of locomotion—how to make an efficient small walker, or how soft-bodied things should move.' Dr Jasmine Nirody
Beyond the implications for evolutionary biology and the study of animal locomotion, the findings may have ramifications for the burgeoning fields of soft and microscale robotics.
By studying how small animals evolved to move across challenging environments, scientists may be able to design robots that can more efficiently squeeze into small spaces or operate at the microscale.
'Tardigrades exhibit robust interlimb coordination across walking speeds and terrains' published by PNAS
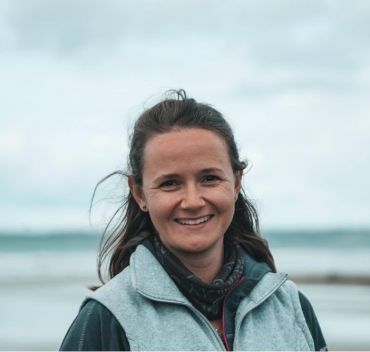
Jack is still actively studying the geology of the lunar surface, and when the Lunar Reconnaissance Orbiter which launched in 2009 took exceptionally high-resolution photographs of the lunar surface, he realised the true significance of some of the samples he had collected.
Rather than representing debris strewn across the Moon during meteorite impacts he realised that the boulders he had sampled, which are part of large-scale lava flows like those we see today in Hawaii, had barely moved since they cooled and crystallized over 3.5 billion years ago.
Prof. Claire Nichols, Associate Professor at the Department of Earth Science, studies planetary magnetic fields, and the Moon’s magnetic field history is particularly curious. Magnetic fields are driven in the dense iron-rich cores of planets by vigorous stirring of the electrically conductive, liquid metal.
Earth’s core is roughly half the radius of the planet, and the outer liquid part drives the bar-magnet-like field we observe around Earth today, responsible for the north and south magnetic poles aligned roughly along the planet’s rotation axis.
‘During the final manned mission to the Moon 49 years ago, Apollo 17 collected rocks from the lunar surface including samples which would turn out to be critical for our study nearly 50 years later.’
The Moon on the other hand has a tiny core, just one seventh of its radius. With such a tiny source of conductive material so far from the planet’s surface (the strength of the magnetic field drops off with distance from source cubed), we would never predict that the Moon generated a significant magnetic field. However, samples from the Apollo missions reveal that the Moon generated an intense magnetic field for at least 2 billion years.
Modellers have tried to simulate how such a strong and long-lived lunar magnetic field could be generated but have struggled to come up with plausible mechanisms. One issue is that observations have only revealed the strength of the magnetic field over time, rather than its geometry and orientation. Both observations are needed to be able to distinguish between the possible physical processes which stirred the molten part of the lunar core.
Almost all Apollo samples collected are loose material scattered across the lunar surface, and their original position and orientation are unknown. So when Jack realised he had collected samples whose original orientation could be recovered, he got in touch with the MIT Paleomagnetism Laboratory, to let us know he had just the samples we had been looking for.
After arriving on Earth, every sample from the Apollo missions was meticulously documented and photographed under lunar lighting conditions. In other words, light is shone onto the samples in such a way to exactly recreate the illumination and shadows when the sample was collected on the lunar surface.
‘The results suggest that the ancient lunar magnetic field had a very similar geometry to the magnetic field around the Earth today, which wasn’t what we had expected.’
We were able to borrow samples from the Johnson Space Center in Houston, Texas which were hammered off the rocky outcrops that Jack had determined must be close to their original position. We used the laboratory photographs to match up all the features with those in the astronaut photographs to work out the exact orientation the samples were in on the lunar surface when they crystallized 3750 million years ago.

Once we knew the orientation of the samples, we demagnetized them in the MIT Paleomagnetism Laboratory using a superconducting rock magnetometer to recover the strength – and for the first time – the direction of the ancient lunar magnetic field.
As previous studies had shown, we found evidence that the lunar magnetic field at this time was very intense – similar to the strength of Earth’s magnetic field today. We were also able to recover how steep the magnetic field was relative to the surface at Camelot Crater, where the samples were collected. This steepness can be used to determine where the magnetic north and south pole were relative to our sample’s location. We found that the magnetic poles were aligned along the present-day spin axis of the Moon (the axis around which the Moon rotates so that we always see the same side of it as it orbits around the Earth).
Our results suggest that the ancient lunar magnetic field had a very similar geometry to the magnetic field around the Earth today. This wasn’t what we had expected; given the strength of the Moon’s magnetic field, calculations show that it cannot have been generated by the same mechanism that drives Earth’s magnetic field. Additionally, because the Moon rotates incredibly slowly compared to the Earth - it rotates just once every 27 days - we wouldn’t expect the magnetic poles to be aligned along the spin axis. Instead, we would expect the magnetic field to be disorganized and have a much more complex pattern of magnetic field lines.
The alignment of the lunar magnetic poles along the spin axis is an important observation for understanding the interior dynamics of the Moon during its early history. Some model predictions have suggested that the mixing of molten iron in the centre of the Moon could have been physically driven by gravitational stirring. The rocky outer part of the Moon, the liquid metallic outer core and the solid metallic inner core all rotate at slightly different angles. This generates friction between the layers that could have generated a magnetic field – and given the alignment with the spin axis – seems the most likely candidate for driving the ancient field.
‘Samples from the Apollo missions reveal that the Moon generated an intense magnetic field for at least 2 billion years.’
The Moon is also thought to have shifted relative to its present-day spin axis over time. In other words, if we could travel billions of years back in time, we would be looking at a slightly different side of the Moon, and features such as craters and lava flows would be at different angles to us. This phenomenon happens when there are large-scale changes to the density distribution of a planet and is referred to as ‘true polar wander’. Independent studies have found that the Moon’s polar ice caps have shifted slightly over time due to this wander, and our prediction for the location of the magnetic north pole is consistent with that shift.
There are still many unanswered questions about the Moon’s geologic history, but our study has shown the power of working on oriented samples from other planets. This single observation at just one location on the lunar surface has significantly improved our understanding of how the ancient lunar magnetic field was generated.
We are about to enter a new dawn of planetary science with oriented samples currently being drilled on the Martian surface and due for return in the next decade, and the Artemis program aiming to send humans back to the Moon by 2024. We have shown the significance of collecting oriented samples collected in geologic context that have not moved since their formation. Our results can guide future sampling strategies so we can understand more about how the interior of the Moon has evolved over time.
The full paper, The palaeoinclination of the ancient lunar magnetic field from an Apollo 17 basalt, was published in Nature Astronomy.
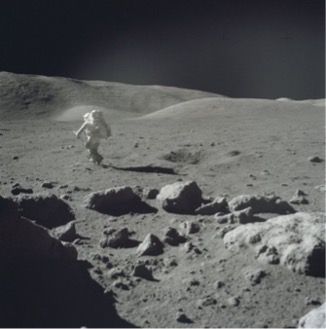
The Moon’s polar regions are home to craters and other depressions that never receive sunlight. Permanently shadowed lunar craters contain water ice but are difficult to image. An AI algorithm now provides sharper images, allowing us to see into them with high resolution for the first time.
Near the lunar north and south poles, the incident sunlight enters the craters and depressions at a very shallow angle and never reaches some of their floors.
Dr. Valentin Bickel, MPS
Today, a group of researchers led by the Max Planck Institute for Solar System Research (MPS) in Germany, supported by the University of Oxford and the NASA Ames Research Center, have taken a closer look at some of these regions and presented the highest-resolution images to date covering 17 such craters in the journal Nature Communications.
Craters of this type could contain frozen water, making them attractive targets for future lunar missions, and the researchers focused further on relatively small and accessible craters surrounded by gentle slopes. Three of the craters have turned out to lie within the just-announced mission area of NASA's Volatiles Investigating Polar Exploration Rover (VIPER), which is scheduled to touch down on the Moon in 2023.
Imaging the interior of permanently shadowed craters is difficult, and efforts so far have relied on long exposure times resulting in smearing and lower resolution. By taking advantage of reflected sunlight from nearby hills and a novel image processing method, the researchers have now produced images at 1-2 meters per pixel, which is at or very close to the best capability of the cameras.
The Moon is a cold, dry desert. Unlike the Earth, it is not surrounded by a protective atmosphere and water which existed during the Moon’s formation has long since evaporated under the influence of solar radiation and escaped into space. Nevertheless, craters and depressions in the polar regions give some reason to hope for limited water resources.
'Near the lunar north and south poles, the incident sunlight enters the craters and depressions at a very shallow angle and never reaches some of their floors', MPS-scientist Dr. Valentin Bickel, first author of the new paper, explains. In this "eternal night," temperatures in some places are so cold that frozen water is expected to have lasted for millions of years. Impacts from comets or asteroids could have delivered it, or it could have been outgassed by volcanic eruptions, or formed by the interaction of the surface with the solar wind.

Measurements of neutron flux and infrared radiation obtained by space probes in recent years indicate the presence of water in these regions. Eventually, NASA’s Lunar Crater Observation and Sensing Satellite (LCROSS) provided direct proof: twelve years ago, the probe fired a projectile into the shadowed south pole crater Cabeus. As later analysis showed, the dust cloud emitted into space contained a considerable amount of water.
'With the help of the new HORUS images, it is now possible to understand the geology of lunar shadowed regions much better than before, Ben Moseley', Oxford University.
However, permanently shadowed regions are not only of scientific interest. If humans are to ever spend extended periods of time on the Moon, naturally occurring water will be a valuable resource - and shadowed craters and depressions will be an important destination.
NASA's uncrewed VIPER rover, for example, will explore the South Pole region in 2023 and enter such craters. To get a precise picture of their topography and geology in advance - for mission planning purposes, for example - images from space probes are indispensable. NASA’s Lunar Reconnaissance Orbiter (LRO) has been providing such images since 2009.
However, capturing images within the deep darkness of permanently shadowed regions is exceptionally difficult; after all, the only sources of light are scattered light, such as that reflecting off the Earth and the surrounding topography, and faint starlight. 'Because the spacecraft is in motion, the LRO images are completely blurred at long exposure times,' explains Ben Moseley of the University of Oxford, a co-author of the study.
At short exposure times, the spatial resolution is much better. However, due to the small amounts of light available, these images are dominated by noise, making it hard to distinguish real geological features. To address this problem, the researchers have developed a machine learning algorithm called HORUS (Hyper-effective nOise Removal U-net Software) that "cleans up" such noisy images.
It uses more than 70,000 LRO calibration images taken on the dark side of the Moon as well as information about camera temperature and the spacecraft's trajectory to distinguish which structures in the image are artifacts and which are real. This way, the researchers can achieve a resolution of about 1-2 meters per pixel, which is five to ten times higher than the resolution of all previously available images.
Using this method, the researchers have now re-evaluated images of 17 shadowed regions from the lunar south pole region which measure between 0.18 and 54 square kilometers in size.
In the resulting images, small geological structures only a few meters across can be discerned much more clearly than before. These structures include boulders or very small craters, which can be found everywhere on the lunar surface. Since the Moon has no atmosphere, very small meteorites repeatedly fall onto its surface and create such mini craters.
Because the spacecraft is in motion, the LRO images are completely blurred at long exposure times, Ben Moseley, Oxford University.
'With the help of the new HORUS images, it is now possible to understand the geology of lunar shadowed regions much better than before,' explains Moseley. For example, the number and shape of the small craters provide information about the age and composition of the surface. It also makes it easier to identify potential obstacles and hazards for rovers or astronauts. In one of the studied craters, located on the Leibnitz Plateau, the researchers discovered a strikingly bright mini-crater.
‘Its comparatively bright color may indicate that this crater is relatively young,’ says Bickel. Because such a fresh scar provides fairly unhindered insight into deeper layers, this site could be an interesting target for future missions, the researchers suggest.
The new images do not provide evidence of frozen water on the surface, such as bright patches. “Some of the regions we've targeted might be slightly too warm," Bickel speculates. It is likely that lunar water does not exist as a clearly visible deposit on the surface at all - instead, it could be intermixed with the regolith and dust, or may be hidden underground.
To address this and other questions, the researchers' next step is to use HORUS to study as many shadowed regions as possible. ‘In the current publication, we wanted to show what our algorithm can do. Now we want to apply it as comprehensively as possible,’ says Bickel.
This work has been enabled by the Frontier Development Lab (FDL.ai). FDL is a co-operative agreement between NASA, the SETI Institute (seti.org) and Trillium Technologies Inc, in partnership with the Luxembourg Space Agency and Google Cloud.
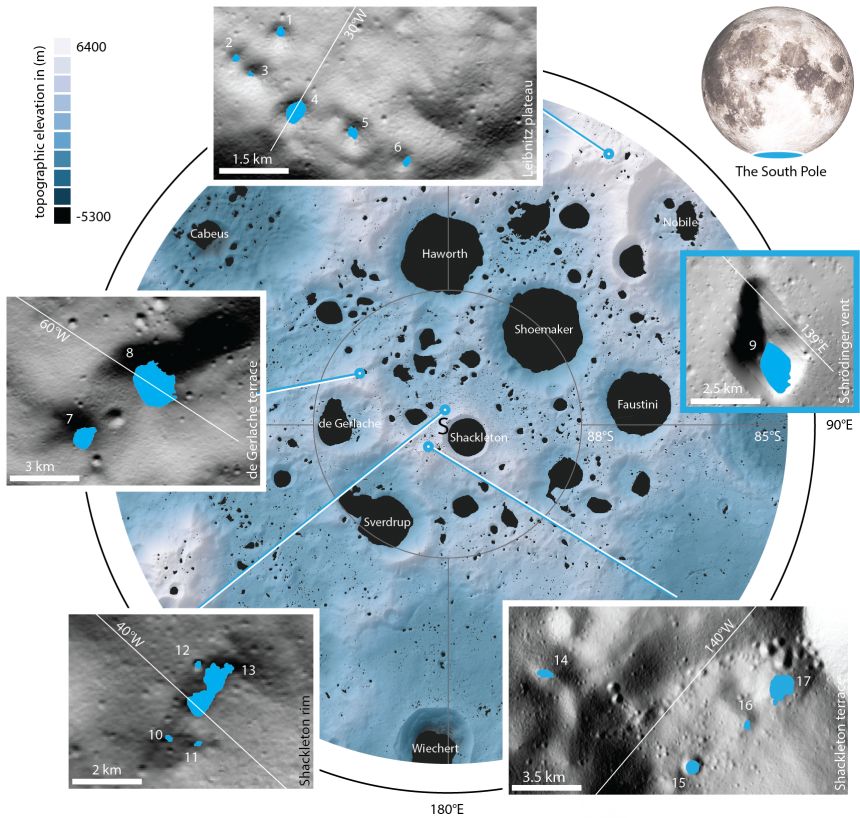
- ‹ previous
- 13 of 248
- next ›